Quantifying COVID-19 Pandemic Spread
Metrics, Mathematics, and Models to Guide Effective Responses
Alison Hill
Assistant Professor
Institute for Computational Medicine
Johns Hopkins University
Sponsored by PSW Science Member Tim Thomas
About the Lecture
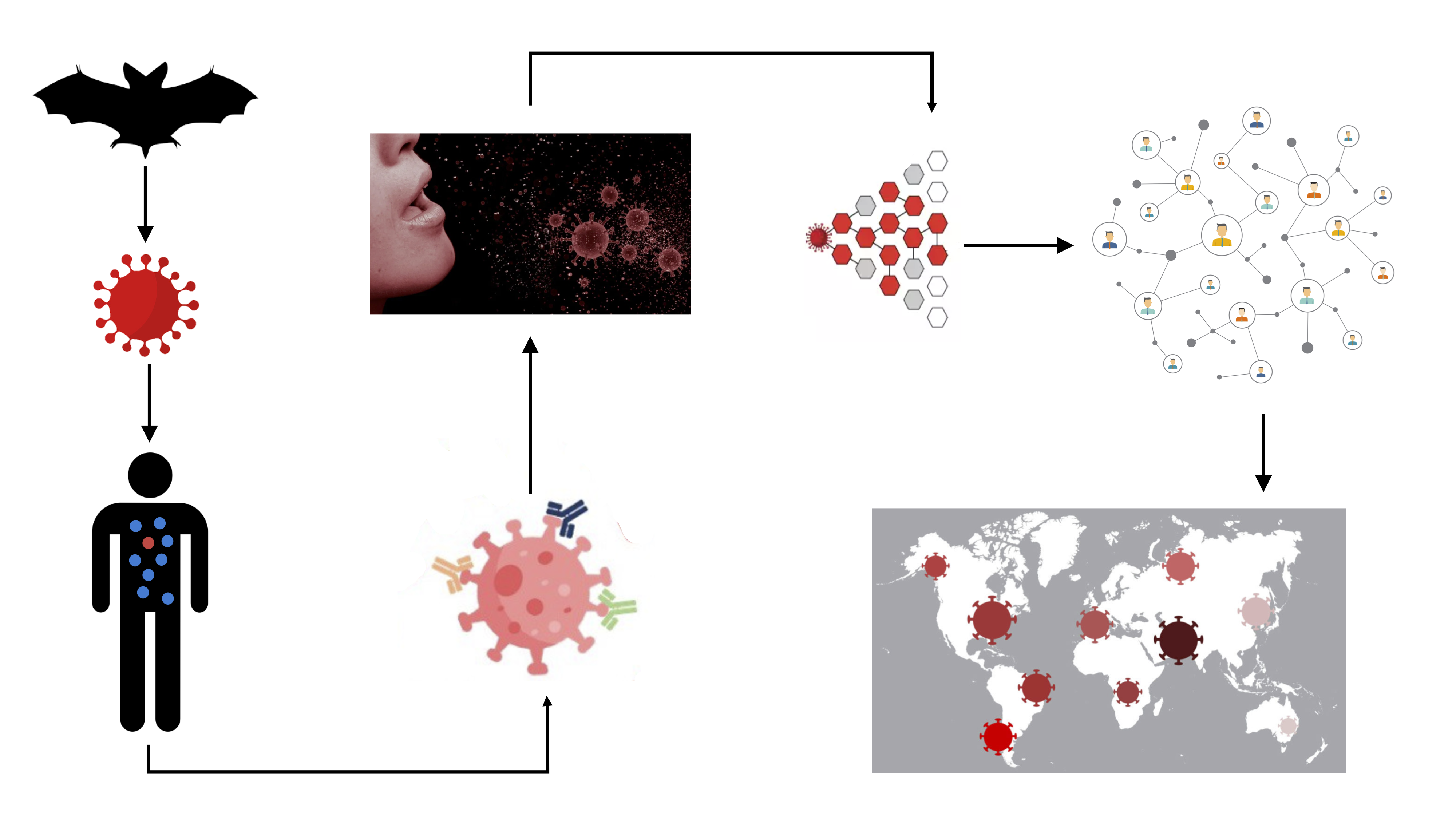
This lecture will discuss the mathematics of infectious disease spread, its use in modeling the spread of diseases, such as COVID-19, and its application in formulating effective public health policies to curtail epidemics and minimize their effects.
Reading references –
A.L. Hill, The math behind epidemics, Physics Today. 73 (2020) 28–34. https://doi.org/10.1063/PT.3.4614.
A. Vespignani, H. Tian, C. Dye, J.O. Lloyd-Smith, R.M. Eggo, M. Shrestha, S.V. Scarpino, B. Gutierrez, M.U.G. Kraemer, J. Wu, K. Leung, G.M. Leung, Modelling COVID-19, Nature Reviews Physics. 2 (2020) 279–281. https://doi.org/10.1038/s42254-020-0178-4.
N.C. Grassly, C. Fraser, Mathematical models of infectious disease transmission, Nat Rev Micro. 6 (2008) 477–487. https://doi.org/10.1038/nrmicro1845.
About the Speaker
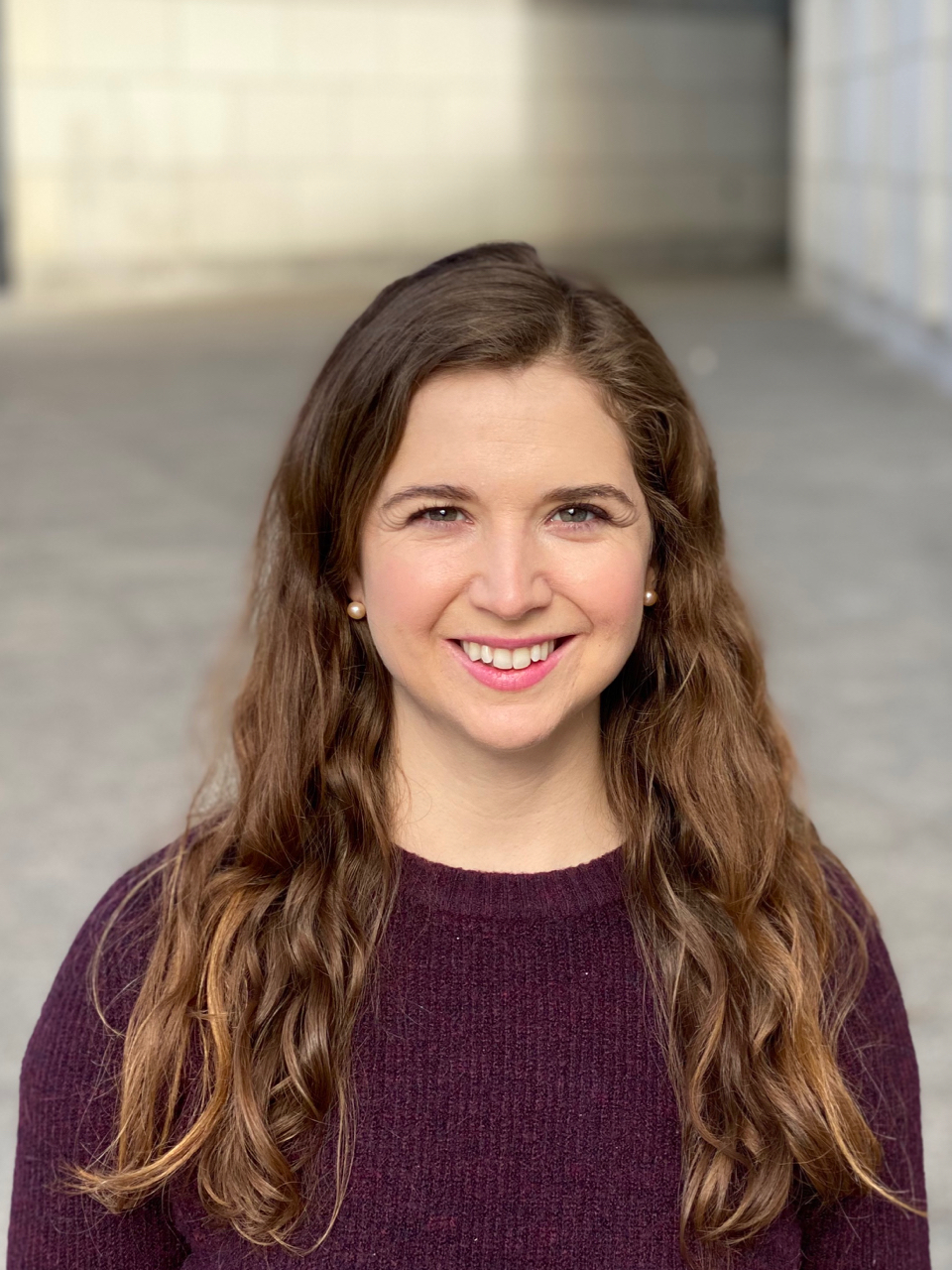
Alison Hill is Assistant Professor in the Institute for Computational Medicine at the Johns Hopkins University. Alison and her team develop mathematical models and computational tools to help understand, predict, and treat infectious diseases, with a particular focus on HIV/AIDS, COVID-19, drug resistant infections, bed bugs infestations, and anti-viral immune responses.
Alison is a recipient of the NIH Director’s Early Independence Award and was selected as one of the World Economic Forum’s Young Scientists. She has written popular science pieces on infectious disease dynamics for PBS Nova and Physics Today, and is a co-chair of the COVID-19 Dynamics & Evolution Meeting and the COVID-19 Data Forum.
Alison earner her BS at Queen’s University, Canada and her PhD jointly through the Harvard-MIT Division of Health Sciences & Technology, Medical Engineering & Medical Physics.
Minutes
On January 22, 2021, by Zoom videoconference broadcast on the PSW Science YouTube channel, President Larry Millstein called the 2,433rd meeting of the Society to order at 8:02 p.m. EST. He announced the order of business and welcomed new members. The Recording Secretary then read the minutes of the previous meeting.
President Millstein then introduced the speaker for the evening, Alison Hill, Assistant Professor in the Institute of Computational Medicine at the Johns Hopkins University. Her lecture was titled, “Quantifying COVID-19 Pandemic Spread: Metrics, Mathematics, and Models to Guide Effective Responses.”
Hill recounted the improbable journey of SARS-CoV-2 from an initial animal host to infecting its first human, then its spread to humans around the globe. To effectively combat the virus, researcher have created mathematical models to better understand each hurdle the virus had to overcome to reach the present state of community spread. The more accurately scientists can understand the emergent dynamics of the viral spread, the better able they will be to perturb them.
Compartmental models are the simplest, most common models in the field of infectious disease dynamics. These models classify individuals into discrete stages of infection and track the transition of individuals between them. An emergent property of this system is the basic reproduction ratio, which describes the average number of secondary infections produced by one single infected individual introduced into an otherwise susceptible population. A ratio less than 1 means infections will decrease. Hill said this ratio is heavily dependent on human behavior and environmental factors.
Early stages of an epidemic often involve exponential infection spread because infected people have not yet limited their interactions with susceptible people. As the availability of susceptible people is reduced, viral spread slows and will eventually die out. In this model, the basic reproduction ratio identifies how many people will need to obtain immunity either through vaccination or recovery from infection to reduce the basic reproduction ration to less than 1. This threshold is called “herd immunity,” and is often exceeded in uncontrolled epidemics.
The simple Susceptible, Infected, Recovered (SIR) model assumes a person becomes contagious soon after they are infected, that immunity is lifelong, and ignores demographic processes such as births, deaths, and migrations. More complex models are required to understand viral spread when these considerations cannot be assumed. For example, the compartmental model for COVID-19 spread needs to consider people who have been exposed to SARS-CoV-2, but who are not yet infectious.
Hill said directly observing viral transmission is inherently biased because people are more likely to notice and observe super-spreader events where an unusually high number of people are infected, and people with known infections are more likely to be limiting their interactions. These two biases can result in direct observations concentrate at the high and low ends of viral spread. For these reasons, epidemiologists tend to prefer indirect methods of estimating viral spread.
Researchers have also created models to estimate disease fatality during an epidemic. The resulting quantity is called infection fatality risk (IFR), or case fatality risk (CFR). Hill said an early mistake in the current pandemic was to estimate the disease’s fatality simply by dividing the number of cases by the number of deaths at a particular time. This method was flawed due to the delay between infection and death and the inability to observe all cases. To obtain a more accurate IFR, researchers needed to follow a cohort of individuals until they all had an outcome.
Early models indicated an uncontrolled SARS-CoV-2 epidemic would quickly overrun health care capacity. Based on those models, public health officials strategized to reduce the basic reproduction ratio by limiting person-to-person interactions, and thus slow viral spread to allow health care systems operating within their capacities. This strategy has been referred to as “flatting the curve.” Hill said that to defeat COVID-19, models show we need to “crush the curve” and reduce the basic reproductive ratio to less than 1 to reduce the number of cases requiring hospitalization to within health care system capacities.
Hill said more accurate viral spread models account for the networked nature of human contact. Researchers learned from the AIDS epidemic that while most infected people infect only a few others, some people infect a significant number of other people. The result is what is called a scale-free, or power law, network. Tracking the SARS epidemic in 2003 showed similar spread. These identified “super-spreaders” are not explained by models that rely on random interactions.
Researchers have created multiple models of idealized networks, including uniform random networks, small-world networks, and heterogeneous networks. The same epidemic models will produce different infection curves and epidemic size depending on the network to which they are applied. Heterogeneous networks tend to result in fewer overall infections.
Hill has focused her SARS-CoV-2 research on household versus external transmission. She said “blunt” lockdown and stay-at-home orders address only external transmission, and do not address intra-household transmission. Hill’s team used census and other survey data to create networks that included connections both within and outside individual households. Running COVID-19 models through those networks, Hill’s team determined that near total intervention of external contacts is required to halt viral spread.
In Hill’s experiments, the intervention outcome depended on the relative role of household versus external contacts. Social-distancing policies were most effective where external contacts contributed either significantly or minimally to transmission. Intermediate transmission by external contacts evaded social-distancing measures and continually seeded new households with infection. Delay to peak epidemic also tended to be longer if household spread continued.
Certain networks, for example social and work networks, are highly-clustered. Hill said clustered adoption of social distancing leads to long times to eliminate infection because pockets exist where the infection can exist for long periods of time without intervention.
An individual’s risk of infection depends on household size and occupation. Large households and households with more workers (and workers with more external contacts) increase individual risks for infection.
Some people have attempted to from “bubbles,” for example merged households or shared childcare arrangements, to minimize external contacts. Hill said “bubble” formations should only be recommended in the context of steadily declining cases. Where infection levels were stabilized, but not declining, in Hill’s models, bubbles could increase the probability of resurgence.
Hill then addressed the effects of eviction on infection spread. In Hill’s models, evicted people can either be re-housed, become unhoused, or most commonly become a member of another household. Increasing household sizes increases the individual infection for each household member. Hill said the result was increased risk for the entire population. Adverse impacts of eviction are more pronounced in models that account for neighborhood disparities, because they create sub-populations where infection persists and lengthens overall epidemic length.
Population crowding determines epidemic shape. Based on data from Chinese cities, independent from total population size, COVID-19 was more intense (sharper curve) in sparser cities and less intense (flatter curve) in crowded cities. Hill said more nuanced models are necessary to understand this counter-intuitive results.
The speaker then answered questions from the online audience. One member asked about whether and how Hill’s models account for viral evolution. Hill said many modelers did not initially account for SARS-CoV-2 mutations. Now that variants have been identified, modelers are attempting to account for evolution by assuming the existence of multiple virus species within their models.
Another member asked how models account for people who are infectious before they experience symptoms. Hill said modelers can disparatize stages of infection or create multi-level models that account for how the virus runs its course through the body. She said the difficulty of accounting for these persons is good reason for frequent testing and contract tracing to disrupt viral spread.
After the question and answer period, President Millstein thanked the speaker, made the usual housekeeping announcements, and invited guests to join the Society. At 10:16 p.m., President Millstein adjourned the meeting.
Temperature in Washington, D.C.: 6° C
Weather: Clear
Concurrent Viewers of the Zoom and YouTube live stream, 95 and views on the PSW Science YouTube and Vimeo channels: 167.
Respectfully submitted,
James Heelan, Recording Secretary
Highlights
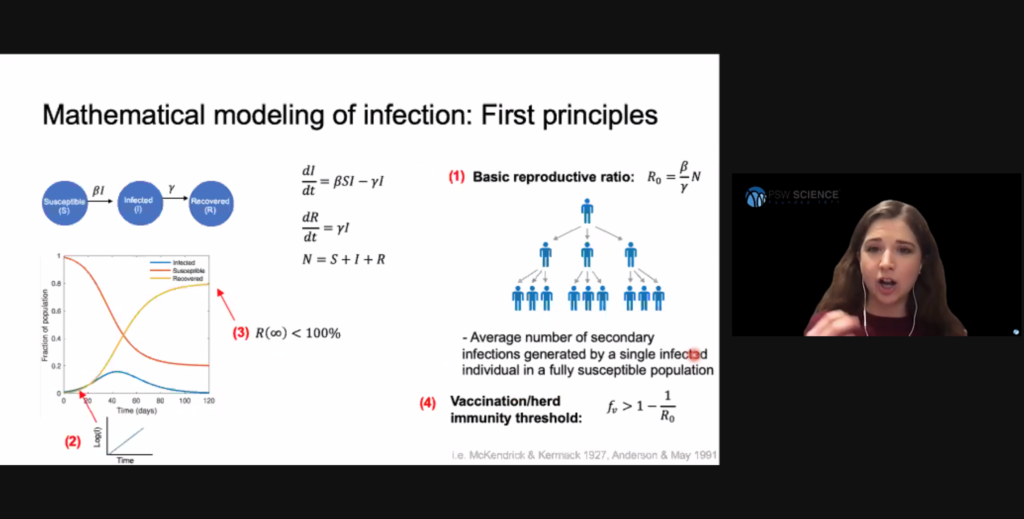
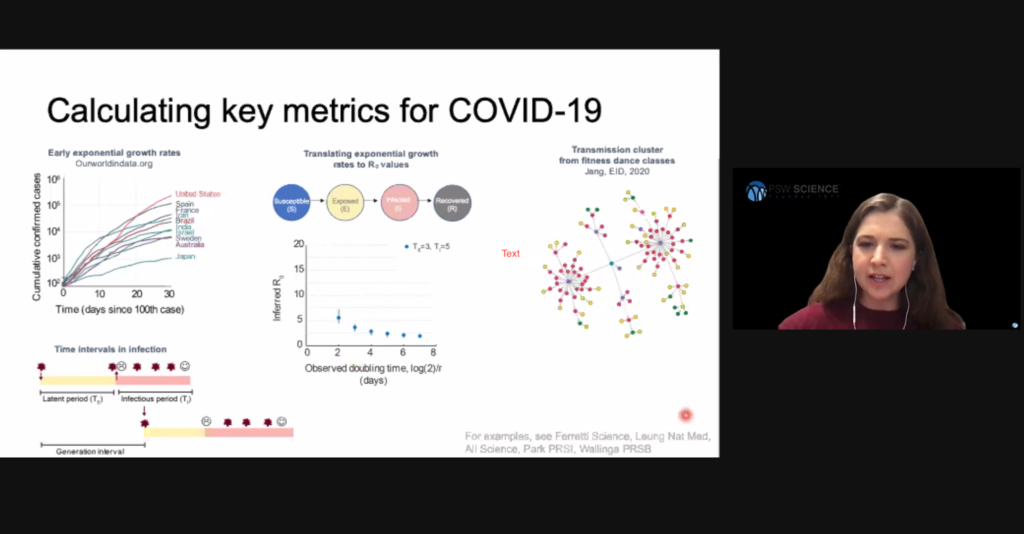
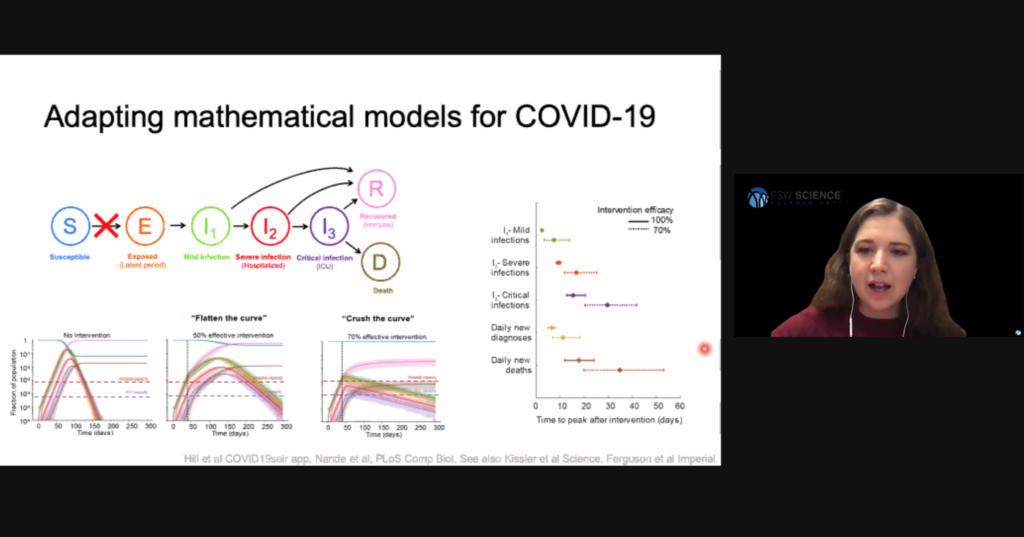
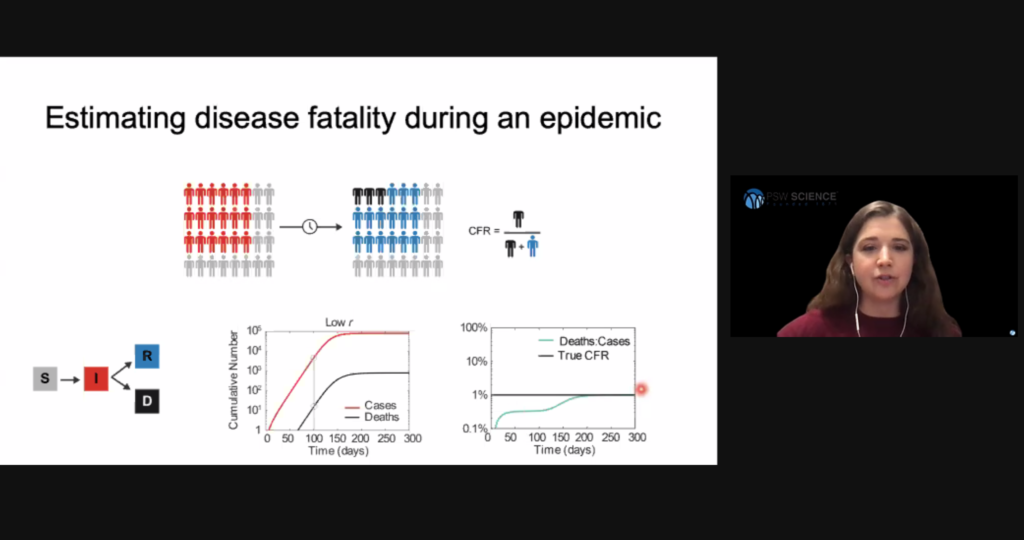