Special Event
LIGO, Gravitational Waves, and Colliding Black Holes
LIGO and the LIGO Result
Speakers from NASA & the University of Maryland
Sponsored by Millen, White, Zelano & Branigan, PC
About the Lecture
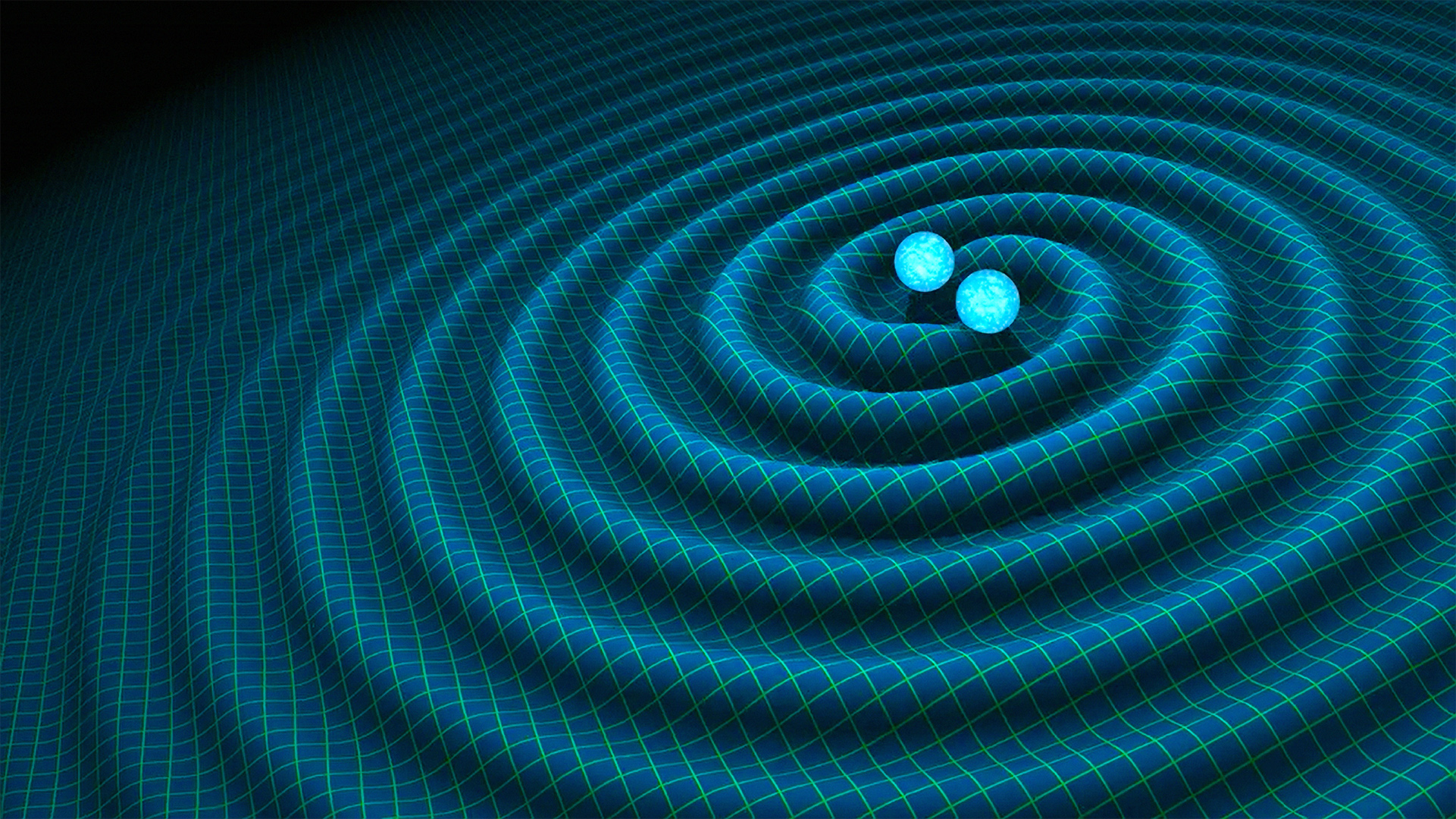
LIGO, Gravitational Waves, and Colliding Black Holes
Three scientists will discuss the recent, historical detection of gravitational waves created by the stupendous collision of two black holes. The discussion will cover the LIGO instruments that detected the waves, the theoretical understanding of gravitational waves, and the cosmic events that can warp the fabric of space-time with sufficient magnitude to propagate across the universe and be detected here on Earth, and the ways in which these events can be detected. Plans for future and more sensitive detectors will be discussed, and how the new field of gravitational wave astronomy is likely to increase our understanding of the universe and influence the fields of fundamental physics and cosmology.
1. The Detection of Gravitational Waves – The Very First Chirp
Peter S. Shawhan
- Associate Professor of Physics
- Department of Physics
- The University of Maryland
The quest to detect gravitational waves — predicted by Albert Einstein’s general theory of relativity, but dismissed by Einstein as an undetectably small effect — began more than 50 years ago at the University of Maryland with modest equipment. Clever ideas, new technologies, and the patient faith of scientists and the National Science Foundation led to the construction of the Laser Interferometer Gravitational-wave Observatory (LIGO), twin sites with exquisitely sensitive detectors capable of measuring extremely tiny changes in the geometry of spacetime itself. But what astrophysical systems exist out there to produce these signals, and how rare are they? The initial LIGO detectors worked as designed but did not yield any clear signal.
A long-awaited upgrade, Advanced LIGO, began collecting science-quality data with even greater sensitivity in September 2015. Almost before we knew it, the detectors captured a breathtaking signal, remarkable both in its clarity and in what it tells us about its source. The scientific team labored in secrecy for months to fully validate the signal and analyze its implications before announcing it to the world on February 11. I will share an insider’s view of the science, the methods, and the findings from this sensational event.
Peter Shawhan attended Washington University in St. Louis as an Arthur Holly Compton Fellow and earned an A.B. (summa cum laude) in 1990. He won an NSF Graduate Research Fellowship and used it at the University of Chicago, where he earned an M.S. in 1992 and Ph.D. in 1999 with a dissertation from the KTeV particle physics experiment at Fermilab. He then went to Caltech as a Millikan Prize Postdoctoral Fellow, switching research fields to work on the relatively young LIGO Project. After that fellowship plus four more years at Caltech as a Senior Scientist, Shawhan joined the University of Maryland faculty in 2006. He is currently Associate Professor and Associate Chair for Graduate Education in the Physics Department, as well as a Fellow of the Joint Space-Science Institute.
Shawhan has held several leadership roles in LIGO, including co-chair of the Burst Analysis Working Group and membership on the LIGO Scientific Collaboration Executive Committee and the LIGO Program Advisory Committee. He serves on the Editorial Board of the journal Classical and Quantum Gravity and is currently the Chair-Elect of the American Physical Society’s Division of Gravitational Physics.
2. The Music of General Relativity – Listening to Black Holes
John Baker
- Astrophysicist
- NASA-Goddard Space Flight Center.
It has now been a century since Einstein published his theory of general relativity and first described gravitational waves. Only much more recently have we come to understand those predictions in sufficient detail to fully interpret the first gravitational-wave observations of merging black holes. The detailed predictions of Einstein’s theory provide the foundation for interpreting our new hearing-like sense of the universe provided by gravitational-wave astronomy.
Black hole mergers, whether they are mergers of the collapsed cores of giant stars or mergers of the gargantuan supermassive black holes at the centers of galaxies, are believed to be the most powerful astronomical cataclysms. The whirling dance of the black holes on their way to merger release as much energy as the light from all the stars in the sky in the form of gravitational-waves. Those waves in turn provide a detailed record of choreography of the merger and thus also reveal the characteristics of the black holes. I will discuss our theoretical understanding of black hole mergers and their predicted gravitational-wave signatures. The observation of GW150914 provides our first direct evidence of these systems and the strong gravity phenomena involved in their mergers. This observation, though, is just our first step toward the richer understanding of gravity and the dynamic world of dark objects in the universe which gravitational-wave astronomy can provide.
John Baker is an astrophysicist in the Gravitational Astrophysics Laboratory at NASA’s Goddard Space Flight Center in Greenbelt, MD. He received his PhD in Physics from the Pennsylvania State University in 1999 for mathematical work on the end-state of black hole mergers. Subsequently he held postdoctoral appointments at the Albert Einstein Institute in Germany where he developed facility with numerical relativity techniques, and at NASA. He joined NASA as a civil servant astrophysicist in 2004. In 2005-6 his team developed computational techniques which finally enabled effective numerical simulations of black hole mergers and computed the first predictions for the gravitational-wave signals from those events. For this work, he later received the John C. Lindsay Memorial Award.
Since that time John has continued to explore black hole merger phenomenology through numerical simulations, recently also including the dynamics of magnetized gasses which may happen to be present during the merger. His work has also expanded to include gravitational- wave data analysis techniques and aspects of gravitational-wave mission design in support of a future space-based gravitational-wave instrument. Currently John serves on NASA’s L3 Study Team helping to explore a role for NASA in the European Space Agency’s future L3 gravitational-wave mission.
3. New Astrophysics: Observing Gravitational Waves Across the EM Spectrum
Julie McEnery
- Astrophysicist and Fermi Project Scientist
- Astrophysics Science Division
- NASA /Goddard Space Flight Center
Gravitational wave observatories are providing us with a spectacular new window on some of the most extreme phenomena in the Universe. To put these new discoveries into context, we conduct coordinated observations with more traditional telescopes from radio to gamma-ray wavebands. Gravitational wave observations provide us with exquisite information about the nature and geometry of the compact objects responsible for producing the gravitational wave signal. Complementary electromagnetic observations can provide us with information about the environment around gravitational wave sources. This is critically important for placing gravitational wave sources within the broader astrophysical context. In this talk, I will describe what we are looking for and why, how we search for electromagnetic counterparts, progress to date and future prospects.
Julie McEnery is the Project Scientist for the Fermi gamma-ray Space Telescope and an astrophysicist in the Astrophysics Science Division of NASA’s Goddard Space Flight Center. She is an Adjunct Professor of Physics at the University of Maryland, Co-Director of the Joint Space Science Institute and a fellow of the American Physical Society.
Prior to joining NASA, Julie worked with ground-based gamma-ray telescopes, which detect radiation produced when gamma rays from deep space strike Earth’s atmosphere. As a graduate student at University College Dublin, she used the Whipple Observatory to make very-high- energy observations of the active galaxy Markarian 421. She later worked at the University of Utah, the University of Wisconsin and Los Alamos National Laboratory on the Milagro gamma-ray observatory, primarily on gamma-ray burst (GRB) observations.
Active galaxies and GRBs remain her main science interests, but she also explores interesting topics in other areas of very high energy astrophysics.
Minutes
President Larry Millstein called the 2363rd meeting of the Society to order at 8:04 p.m. He announced the order of business and welcomed new members. The minutes of the previous meeting were read and approved. President Millstein then introduced the speakers for the evening, Dr. Peter S. Shawhan, Associate Professor of Physics at the University of Maryland; Dr. John G. Baker, Astrophysicist at the NASA/Goddard Space Flight Center; and Julie McEnery, ([pronounced “Mc-N-ry” or “McHenry”, basically]) Astrophysicist and Fermi Project Scientist at the NASA/Goddard Space Flight Center. Their lecture was titled “LIGO, Gravitational Waves, and Colliding Black Holes”.
Dr. Shawhan began by explaining that General Relativity describes gravity as a consequence of the curvature of space time caused by the presence of mass. Mass in turn responds to this curvature. The effect of gravity can be carried as waves, which manifest as distortions in the geometry of spacetime—stretching and squeezing as these waves pass through an object.
All objects with mass affect spacetime, and so a massive object in motion creates the distortion over time that we call a gravitational wave. Compact, massive objects—such as neutron stars or black holes—that are orbiting each other are a good source of gravitational waves because the changing mass configuration creates a pattern of distortion each time they orbit.
Because the amplitude of the distortion effect falls inversely proportional to the distance from the source, any distortion is quite small when it reaches us. The typical distortion that we expect to see on Earth is 1 in 10-21. In other words, the diameter of the Earth would change by 10-14 meters, or a few times the width of a proton. Einstein predicted the effect, but had no expectation it would ever be observed.
The Large Interferometer Gravitational-Wave Observatory, or LIGO, involves two L-shaped observatories, one in Hanford, Washington and one in Livingston, Louisisana. Each LIGO detector consists of a pair of 4km tubes, each containing a long vacuum chamber. A single laser beam is split and directed down each tunnel, to be reflected by a mirror at the far end. When they arrive back at the LIGO detector, these separate beams are recombined. When recombined, the phases of the waves should match up and the light output remain the same. Distortions in space time manifest as discrepancies in the arrival time of light waves, which would knock the beams out of phase. Thus, LIGO can detect gravity by measuring an increase or decrease in the light intensity caused by constructive or destructive interference between the mismatched phases of the laser beams.
In September 2015, when LIGO was activated for a test run, it captured a split-second “Coherent Wave Burst Event”. The rising frequency of the signal was consistent with the pattern of a binary black hole merger event, in which two black holes would orbit faster and faster until they merged.
The event radiated energy three times the entire mass of our sun. In that moment the power output was fifty times the total output of all the stars in the universe. Dr. Shawhan noted that once LIGO is at full strength, it will be able to observe events over a volume 27 times larger than this early run, resulting, hopefully, in many more events and much better understanding.
Dr. Baker explained that if two black holes exist in proximity to one another, the gravitational waves generated by their orbit sap energy and angular momentum away from the system, causing them to drift closer and thus spin faster, which generates still greater gravitational waves. This feedback loop accelerates until the black holes merge. The speed of this decay scales directly with the mass of the black holes: The faster the system evolves, the more massive it must be. Once we have the mass, we can work backward from the observed amplitude to determine the distance. We can even infer the relative masses of the two black holes by analyzing any asymmetry in the gravitational wave signal
Dr. Baker noted that higher frequency gravitational wave signals are easier to detect, but they arising from (relatively) unusual events. There are many low frequency events that have a great deal to tell us about the universe but must be observed at greater sensitivity and on a longer time scale. Future space-based LIGO programs will extend our capabilities dramatically.
Dr. McEnery explained that, about once a day, we detect a gamma ray burst, which for a brief period outshines everything else in the gamma ray sky. These phenomena was first discovered in the 1960s by satellites designed to detect nuclear tests, but for 30 years we didn’t know what they were or even where they were.
Because they were so intense, it was assumed that they originated relatively close by, on a cosmic scale. In 1997, we were able to measure the redshift of the x-ray afterglow of a burst and determine that they in fact originated very far away…and therefore had to be one of the most energetic phenomenon in the known universe.
Short gamma ray bursts are hypothesized to be produced by the merger of two massive objects, the same processes that LIGO detects. Thus, if LIGO sees an event at the same time we observe a gamma ray burst, this will provide empirical confirmation of this 50 year old mystery.
Dr. McEnery noted that co-observations between gamma ray burst observatories and LIGO will provide other valuable data as well, because gamma ray bursts can be localized much more accurately than gravitational waves, allowing cosmologists to fill in critical context about the LIGO data.
After the conclusion of the talk, President Millstein invited questions from the audience.
One questioner asked whether gravity waves were expected to display light-like behaviors such as being scattered or refracted by concentrations of mass. This would be the case if gravity was conveyed by gravitons, but the early LIGO results do not indicate such distortion, which limits the potential for a particle-like model of gravity.
Another questioner asked whether there was a delay between gravitational observations and gamma ray burst observations, and whether this delay could be used alert radio astronomy telescopes to catch more gamma ray bursts. Dr. McEnery indicated that some pf the visible and X-rays associated with gamma ray bursts would likely develop discernible delay, and thus telescopes could potentially be positioned to observed these emissions after receiving notification from LIGO. Telescopes would need to slew fast, however, because the delay would be mere minutes.
After the question and answer period, President Millstein thanked the speaker, made the usual housekeeping announcements, and invited guests to join the Society. At 9:55 p.m., President Millstein adjourned the 2363th meeting of the Society to the social hour.
Attendance: 146
The weather: Cloudy
The temperature: 11°C
Respectfully submitted,
Preston Thomas
External Communications Director