Studies of Human Disease
At the Intersection of Engineering, Natural Science, and Medicine
Subra Suresh
Director
National Science Foundation
About the Lecture
This presentation will provide an overview of some recent research as well as opportunities for new discoveries into human health and diseases through studies at the intersections of different disciplines. Specific examples of such multidisciplinary approaches will be presented in the context of human malaria, hereditary blood disorders and cancer. Wherever appropriate, discussions of state-of-the-art experimental results will be accompanied by multi-scale computational simulations and experiments. Implications for multidisciplinary approaches that rely on recent developments in engineering, biophysics, genetics and biochemistry will be examined in light of fundamental mechanistic understanding, diagnostics and therapeutics.
About the Speaker
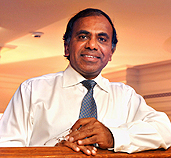
DR. SUBRA SURESH is the Director of the National Science Foundation (NSF) and oversees this unique federal agency’s annual investment of $7 billion in fundamental research and education in science, technology, engineering, and mathematics. NSF is the only U.S. government agency dedicated to advancing basic research and education across the entire spectrum of disciplines and fields and at all educational levels. The goal is to keep the United States at the forefront of science and engineering, empower future generations of scientists and engineers, foster economic growth and innovation, and improve the quality of life for all Americans. Dr. Suresh pioneered research to uncover the mechanical properties of materials. His most recent research has focused on the biomechanics of red blood cells and the changes wrought in their biomechanical properties by disease and genetic disorders. In 2006, Technology Review named his nanobiomechanics work as one of the top-ten technologies that “will have a significant impact on business, medicine or culture.” Dr. Suresh earned a BTech from the Indian Institute of Technology, an MS from Iowa State University, and a ScD from the Massachusetts Institute of Technology (MIT) in 1981. Before becoming NSF Director, he was Dean of the Engineering School and Vannevar Bush Professor of Engineering at MIT. He joined MIT in 1993 as R.P. Simmons Professor of Materials Science and Engineering where he has held joint faculty appointments in several departments. Prior to joining MIT faculty, he held appointments at the University of California-Berkeley, Lawrence Berkeley National Lab, and Brown University. He has mentored more than 100 engineers and scientists and has been a strong and effective champion of increasing diversity at MIT. He has authored more than 230 research articles, several widely used texts, including Fatigue of Materials, and he is a co-inventor in more than 22 patent applications. He has been a consultant to a wide range of corporations, research laboratories, non-profit organizations and governments and served on several international study groups and advisory panels. Dr. Suresh is a member of the National Academy of Engineering, American Academy of Arts and Sciences, Indian Academy of Engineering, Indian Academy of Sciences, Spanish Royal Academy of Sciences, German National Academy of Sciences, and Academy of Sciences of the Developing World. He is a fellow of the American Society of Materials International, the Materials Research Society, the American Society of Mechanical Engineers, the American Ceramic Society, the Indian Institute of Metals, and the Materials Research Society of India. He has received many awards, including, recently, the Padma Shri Award given by the President of India, General President’s Award of the Indian Science Congress, Eringen Medal of the Society of Engineering Science, European Materials Medal, and Acta Materialia Gold Medal. Dr. Suresh has received honorary doctorate degrees from the Swedish Royal Institute of Technology and the Polytechnic University of Madrid. Dr. Suresh has been married to his wife Mary since 1986, and they have two daughters, Nina and Meera.
Minutes
President Taylor called the 2284th meeting of the Philosophical Meeting to order at 8:18 PM on April 8, 2011 in the Powell Auditorium of the Cosmos Club, Washington, DC. She announced the order of business and introduced newly elected members. President Elect Ingersoll then read the minutes of the 2283rd meeting. There were no comments on the minutes and they were approved by voice vote.
Ms. Taylor introduced the speaker, Subra Suresh, D.Sc., Director of the National Science Foundation and his talk, “Studies of Human Diseases at the Intersections of Engineering, Natural Sciences, and Medicine.”
There have been several major revolutions in the last decade that—in combination—promise dramatic advances in our understanding of disease and improvements in health care outcome. Advances in physics, particularly for manipulating and measuring things on the nano-scale level; molecular biology and genomics; microfabrication and microfluidics; computational tools (both hardware and software); and the development of bioengineering as an integrated discipline with established departments at all major U.S. research universities are among these revolutions.
Advances engendered by combining these revolutionary technologies can be seen in recent work on malaria and red blood cells (RBCs), inherited blood disorders, and cancer, relating to the fundamental question: How are disease states influenced by cellular- and sub-cellular phenomena?
Malaria is caused primarily by Plasmodium falciparum and Plasmodium vivax, the former being responsible for most fatalities. The parasites are carried by mosquitoes, enter humans’ blood through mosquito bites, and multiply in red blood cells. The body produces 300,000–500,000 RBCs every second. They are shaped to maximize surface-to-volume ratio. They are 8 µm in diameter and have to pass through a capillary only 2 µm in diameter to deliver oxygen to the human brain. To do so, RBCs must elongate and stretch, repeatedly, perhaps more than 1,000,000 times over the normal RBC lifetime. Loss of RBC flexibility leads to disease, and the ability to detect changes in flexibility can be used to develop diagnostic tests and to link specific proteins and genes to mechanical properties of RBCs.
However, it has not been possible until recently to directly measure the flexibility of RBCs. The development of Light Tweezers—a pure-physics tool that can measure forces of a few pico-newtons—has made this possible. (Energy Secretary Chu and his colleagues won a Nobel Prize for developing the technique.) Measurements made with this tool have shown both that healthy RBCs are very flexible and that their flexibility is reduced 100-fold—much more than biologists had thought—when they are infected with the malaria parasite. The loss of flexibility scales with disease progression, and flexibility measurements might one day serve to stage disease to help determine appropriate drug dosage.
Another mechanical aspect of disease is cell vibration. It has been known for more than 100 years that cell membranes fluctuate naturally; but, the displacements are so small—nanometers—and high frequency—milliseconds—that it has not been possible to measure them. Using recent advances in laser interference spectroscopy—again, pure physics—it has become possible to measure the vibrations of living cells in liquid media without physical contact with the cell.
A laser beam passed through an RBC undergoes a phase shift due to differences in refractive indices of medium and cell. The phase shift depends, in part, on the thickness of the cell, as shown by the phase-shift equation. Changes in cell thickness cause corresponding changes in the phase shift, revealing changes in the cell’s vibration. A striking false-color image of an RBC highlighted its vibrational activity. It turns out that cells—even ones without nuclei—resonate freely. This image of malarial cells shows that the normal vibrational pattern is disrupted—becoming inhomogeneous and greatly attenuated over parts of the cell. The effect increases with time after infection. As infected cells lose their flexibility, their vibrations are damped and disrupted.
It has become possible to study the underlying mechanisms of topological and mechanical aspects of cells, as well, by combining revolutionary physics techniques such as those just described, with equally revolutionary advances in genetics and gene technology. For instance, it had been thought for some time that the plasmodium RESA [Ring-infected erythrocyte surface antigen] protein causes infected cells to stiffen, but, it had not been possible to prove this experimentally. The sequencing of the plasmodium genome in 2004 opened the way to make RESA knock-out mutants and to see what happens when cells are infected with plasmodia that cannot express the RESA protein. Cells infected with RESA-expressing plasmodia are two- to three-times stiffer than normal cells, and the stiffness increases with temperature. Cells infected with the RESA knock-out plasmodia were not appreciably stiffer than normal cells, and their stiffness did not increase with temperature. These results open up new avenues for developing therapeutic agents by targeting RESA activity.
Revolutionary developments in microfluidics and microelectronics have provided another new and powerful way of understanding biology and disease. The flow of cells that cannot be observed in vivo, for instance, can now be studied dynamically in microfluidic systems in vitro. The flow of RBCs through the two-micrometer diameter blood vessels that supply the brain can be studied using such devices. A video shows RBCs traversing a two-micrometer constriction in a microfluidic cell. Healthy cells flexibly pass through—elongating and thinning to enter and traverse the channel. Cells infected with the malaria parasite are too rigid to do so. They clog at the entryway and cut off the passage of uninfected cells.
Microfluidic flow units hold promise as simple portable, disposable, inexpensive diagnostic devices to separate healthy and infected cells, determine if malaria is present and measure the parasitic load. Several prototype devices have been built by students at MIT: miniature obstacle courses for flowing cells made of diamond shapes, forward-facing triangles or rear-facing triangles. A video shows RBCs flowing through the devices. Their speed is diagnostic of whether blood cells are healthy or diseased. Another video showed that the courses can separate young from old RBC. There is promise that this type of device will be able to determine if cells are infected with P. falciparum—which is often fatal—or P. vivax, which is more benign. Vivax only infects young RBCs, whereas falciparum infects RBCs of all ages.
The revolution in 3-D computer simulation technology also is leading to advances in biology and medicine. These advances are making it possible, for instance, to understand topological and rheological properties of RBCs and how they are altered in blood cell disorders such as Sickle Cell Anemia, Spherocytosis, and Ovalocytosis. An initial question is: Why are healthy RBCs shaped the way they are? Molecular biology has shown that their shape is determined by a skeleton network of about 80,000 spectrin molecules. Recently developed advanced computational capabilities have made it possible to carry out energy minimization calculations on the complete spectrin network of an RBC—all 80,000 molecules. When the stiffness, curvature, and bending modulus of spectrin are correct, the minimization calculation gives the right shape. A video showed the calculation—starting with a sphere made up of 80,000 triangularly coordinated spectrin molecules—progress in stages to the indented discoid shape of an RBC. Nature got this shape for a particular engineering reason, and the latest tools allow us to understand the connection between mechanical engineering and cell biology.
Simulations using dissipative particle dynamics methods developed in the past ten years can now be combined with data from in vitro microfluids experiments to understand cell flows in capillaries. A video shows actual flows of RBCs in a microfluidic device and the results of a computer modeling simulation of RBCs flowing in the same (virtual) channel. Behavior of the RBCs in the simulation resemble their actual flow in the microfluidic channel. However, neither accounts for the effects of endothelial cell linings on flow in real blood cells. Efforts are under way to produce more realistic devices.
Despite their current limitations, these tools are quite powerful, and they have been used to model RBC micro-circulation and splenic clearance, and the results have been compared with blood flow in isolated perfused human spleens.
A similar interdisciplinary approach has been used to study leukostasis resulting from the accumulation of leukocytes following chemotherapy in patients with cancer. The accumulation can be life threatening. Possibly, it is caused by increased stiffness and cytoadherence of circulating leukemia cells that have been killed by the chemotheraptic agent. Dead cells are 30 times as stiff as healthy cells. The increased stiffness involves actin polymerization and might be prevented by a polymerization inhibitor. Preliminary experiments showed that stiffening caused by daunorubicin can be prevented by the inhibitor cytochalasin D, providing another very early example of how the confluence of new tools from mechanical engineering, computer science, and cell and molecular biology are providing new insights into the mechanisms of health and disease and new avenues to better treatments.
And we are just at the beginning. These tools and the interdisciplinary approach they enable will provide fundamental insights into biological mechanisms that will lead to new and better diagnostics, therapeutic agents and ways of treating disease.
President Taylor then invited questions.
Q&A
Years ago Sickle patients were encouraged to be slightly anemic because there was no drug to reduce stiffness of the RBCs. Has this problem be solved. Not yet, he said. Urea is used today. But we have better in vitro models of sickling, so hopefully….
Is there a connection between aspirin and RBC stiffness? No systematic study of this has been done. But there are some initial results from isolated studies showing the effects on RBC stiffness of some factors such as athletic activity. The tools to study this are relatively new as yet and the community of scientists using them is just beginning to grow.
When RBCs goes through a narrow capillary – does the surface area change? And does the energy change when cells fill the capillary? We cannot tell yet. The chemistry is not right in the in vitro models, because the channels are not lined by endothelial cells. Experiments are on-going to do this and to study this question. And there is a lot to learn as yet. The one movie I showed has a wealth of information on how cells change shape and recover. It takes time to analyze it all.
Forty two years ago I gave a speech on a program for analyzing tRNA folding. Does that mean that I am a pioneer in this, then? Yes.
How often can cells pass through capillaries? In nature 1,000,000 or more. But in vitro they die before you can exhaust them.
Do cells generally get stiffer as they get older? Yes, apparently.
Can the devices you showed be used to separate say stem cells from mature cells? Good question. Even for large populations their are correlations but not direct enough at this time for that sort of application
Could you imagine injecting energy into cells at their resonant energies to change them or to kill diseased cells selective? Don’t think so; but, it’s an interesting line of thought. But these tools can augment existing methods.
Drexler published book on Nanotechnology in the ‘80s using a combination of classical and quantum effects to describe nanoscale phenomena. Are we still in this hybridized state or do we now have a fully quantum mechanical description? For some things – like stiffness – the classical is fine. For others – like molecular dynamics – a quantum mechanical treatment is required.
Will nanotechnology help with the economy? Since NSF funded nanotechnology it knows of 175 start ups interfaced with many companies. By and large nanotechnology offers many great opportunities.
After the Q&A session, Ms. Taylor presented a plaque commemorating the occasion. she made the usual housekeeping announcements, he invited visitors to apply for membership, she announced the Henry Dinner speaker, Debra Elmegreen, Ph.D. and the next meeting, and she then adjourned the 2,284th meeting to the social hour.
Attendance: 64
The weather: Rainy, overcast
The temperature: 6°C
Respectfully submitted,
Larry S. Millstein,
Program Chair