The Ocean Floor
What We Don't Know and Why It Matters
Walter H. F. Smith
NOAA Laboratory for Satellite Altimetry
About the Lecture
The recent collision of the USS San Francisco with an uncharted seamount reminds us that only a few percent of the ocean floor has been thoroughly mapped. Better charting and mapping is needed for navigation, to assess tsunami hazards, and to understand the ocean’s role in climate change. This talk will review the state of knowledge of global ocean floor topography by conventional and space-based techniques, and show what can be gained from a new low-cost space mission. Additional information about “Bathymetry from Space” may be found at Oceanography Magazine, Vol. 17, No. 1
About the Speaker
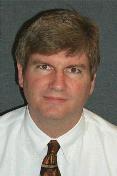
WALTER H. F. SMITH is a Geophysicist in the National Oceanic and Atmospheric Administration’s Laboratory for Satellite Altimetry and chairman of the scientific and technical sub-committee of GEBCO, the international and intergovernmental committee for the General Bathymetric Charts of the Oceans. Smith earned a B. Sc. at the University of Southern California, M.A., M. Phil., and Ph. D. degrees at Columbia University, and was a post-doctoral fellow at the Scripps Institution of Oceanography before joining NOAA. He has published research on ocean floor topography, the Earth’s gravity field, the thermal history of the Earth, and the lubrication of plate tectonics, and is the co-developer of free software for data analysis and mapping: The Generic Mapping Tools He is also a husband and father, sings professionally, and enjoys cooking and sailing.
Minutes
President Robert Hershey called the 2190th meeting to order at 8:20 pm March 25, 2005. The minutes of the 2189th meeting were read and approved.
Mr. Hershey introduced the main speaker of the evening, Mr. Walter H. F. Smith of the National Oceanic and Atmospheric Administration. Mr. Smith spoke on “The Ocean Floor: What We Don't Know and Why It Matters.”
In 1903, a Harvard scientist noted that we had better maps of the moon than of the earth's surface. After the lunar mapping for the Apollo program, A. F. Spilhaus, Sr. told Congress that “we know less about the ocean's bottom than we do about the moon's behind.” Even though polls say the public prefers spending on ocean exploration two to one over space exploration, the situation has not improved. We can now report that the Moon, Mars, Venus, and some asteroids are better mapped than is the ocean's floor.
To put our level of knowledge in perspective, we must consider why it matters.
The tsunami of December 26, 2004 is fresh in our minds. We know tsunami waves may not take a straight path, so predicting arrival is tricky; that the first wave may be less devastating than a later reflected wave; and that nearby points on the coast may see drastically different wave heights. The largest propagation effect is due to seamounts, particularly the submergence depth of their peaks. We've done a poor job to date of finding seamounts. This limits our ability to provide accurate warnings, even with the ongoing improvements in the Pacific tsunami monitoring system.
As computer models of ocean currents advance, they become very sensitive to bottom conditions. For example, one model predicted that the western Pacific current should intrude into the strait between Luzon and Taiwan, although the actual current flows past with little intrusion. When the modelers arbitrarily reduced the depth in a small area, the model became much more accurate. Newer maps showed newly found seamounts in that area. Ocean current models are important in many fields, such as fish migration studies, pollution transport prediction, and search and rescue operations.
A recent, very important advancement in oceanography has been measurement of vertical mixing, which counteracts the oceans natural stratification. Previously, it was believed that tidal energy was dissipated in shallow water. We now know that one-third of the energy is dissipated in deep ocean basins. Bottom roughness transfers energy from tidal flow into turbulence, which mixes the water column and dissipates the energy as heat. This process involves bottom features with spatial wavelengths shorter than 100 km. The vertical roughness may be on the order of 100-150 m.
Models that include variable mixing due to variable bottom roughness produce very different deep flow patterns than models with uniform mixing. In turn, that completely changes the climatically-important transport of heat.
In January of this year, the submarine USS San Francisco collided at high speed with an uncharted obstruction while submerged. One person was killed and nearly the entire crew was injured. If the submarine is removed from service, as has been suggested, the loss would exceed a billion dollars.
So how do we measure the ocean bottom? There are two ways to do this.
Echo sounders provide the most detailed bottom maps, with a pixel size of ~100 m2 and a swath up to twice the depth of the water. The data is concentrated near shipping lanes. If we overlay the tracks from a representative part of the southern oceans on the United States, the track spacing is comparable to the interstate highway system. A map created without leaving the interstate highway system would not be very useful for understanding the U.S. climate, resources, and habitat.
Mapping the ocean floor using satellites is two orders of magnitude faster and cheaper than echo sounding. Satellites in non-repeating orbits can create a very dense array of ground tracks in a few years.
Satellite based hyperspectral techniques can make direct bottom measurements, but only in shallow water.
For most of the ocean, the only general technique is to measure the gravitational tilting of the ocean surface caused by the ocean floor topography's mass. The available echo sounding data (sparse as it is) is then correlated with the tilt data to produce depth information.
The correlation filter is complex, because it must include the effect of the crust's mechanical strength upon the longer wavelengths (tens of kilometers). At wavelengths shorter than the ocean depth, the inverse-squared law attenuates the tilt magnitude, requiring very low signal to noise ratio for useful measurements. The sediment depth must also be estimated.
Comparison of the satellite data with the echo sounding measurements and existing charts reveals just how little we really know. Charts may show fault lines that were interpolated to explain disagreements between two sets of data, and changes in sea floor roughness have been traced to transitions from one cartographer's work to another's.
Another way to test the data is to count seamounts. Plotting the heights of known seamounts against their numbers on a log-log plot produces a strong correlation down to a specific size, below which the number drops off sharply. If this correlation holds true for smaller seamounts, as we expect, the break in the data can be interpreted as a detection limit for the current methods. It also suggests that doubling the detection sensitivity would reveal seventeen times as many seamounts as are now known.
Comparison of the existing charts and the existing satellite gravimetric data with new echo surveys indicates the satellite data is about 70% correct, with an RMS error of about 132 m. The charts prove to be about 70% wrong.
So what more can we learn about the sea floor by using this technique? The abyssal hills, with heights of a few tens of meters, are tantalizingly close to our reach. We haven't found most of the seamounts. An overlay of the satellite data on the area of the USS San Francisco collision indicates a peak a few kilometers from the collision site and to a prior report of discolored water. Note that this is a 1/r2-weighted center of mass, not necessarily the top of a seamount.
Can a new satellite mission do better? The answer is yes. Delay Doppler radar (a synthetic aperture radar technique) should be able to measure sea surface tilt to about one microradian. This accuracy is close to the limit permitted by external noise sources, and will resolve the sea floor roughness down to the limit beyond which statistical extrapolation is most appropriate.
The cost of a new satellite mission is on the order of $0.1 Billion, as opposed to the $1 Billion cost of the USS San Francisco grounding, or the $10 Billion cost of a complete hydrographic survey of the oceans.
The speaker then kindly answered questions from the floor.
Mr. Hershey thanked the speaker, announced the next meeting and made the parking announcement. He invited and encouraged people to join the Society, and adjourned the 2189th meeting at 9:40 pm to the social hour.
Attendance: 51
Temperature: 8° C
Weather: Light Rain
Respectfully submitted,
William Saalbach
Acting Recording Secretary