Improving the Tick-Tock of the Atomic Clock
Telling Time to an Accuracy of One Second in the Lifetime of the Universe
Andrew Ludlow
Project Leader, Time and Frequency Division
National Institute of Standards and Technology
About the Lecture
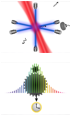
Modern atomic clocks allow us to measure time more precisely than any other physical quantity. This remarkable timekeeping capability lies at the heart of many important everyday technologies, such as the communications networks that support the internet, and the global positioning and navigation systems we fondly refer to as GPS. Time measurements with current state-of-the-art precision and accuracy levels can be leveraged as sensitive probes into the fundamental laws of science. They can be used to test Einstein’s theory of relativity, to search for variations in the fundamental constants of nature, and to search for dark matter. Next-generation timekeepers hold promise for new measurement capabilities and applications, such as gravity sensing for geodetic applications. The most advanced atomic clocks being developed today are known as optical clocks. One type of optical clock, the optical lattice clock, has been rapidly improved since its first inception one decade ago and is the focus of intense research and development worldwide. These clocks will allow us to measure time with an accuracy of one part in 1018, equivalent to about one second in roughly 14 billion years, the age of the universe. These clocks will be broadly discussed, together with recent efforts at NIST towards operating such a clock at the quantum-mechanical limit, and a brief survey of advanced international timekeeping networks and their scientific impact.
About the Speaker

Andrew Ludlow is a physicist and project leader in the Time and Frequency Division of the National Institute of Standards and Technology (NIST) in Boulder, Colorado. His main research activities include the development of optical atomic clocks, cold atom systems and quantum metrology, and ultrastable optical sources and laser interferometry. He currently leads research and development of the ytterbium optical lattice clock at NIST. He has been an author on more than fifty scientific journal articles in the field of atomic clocks. He serves on a variety of technical, scientific, and editorial committees. Among other awards, he received the DAMOP Thesis Prize of the Atomic, Molecular, and Optical Physics Division of the American Physical Society for his doctoral work, Department of Commerce Gold Medal Award, the Young Scientist Award of the European Frequency and Time Forum, and the Rocky Mountain Eagle Award for Scientific Achievement. Andrew earned a BS in Physics from Brigham Young University and a PhD n Physics from the University of Colorado, working at JILA Institute of Physical Sciences run jointly by CU and NIST. After completing his doctoral work at CU he was a National Research Council Postdoctoral Fellow at NIST, where he has since become a research physicist and project leader.
Minutes
President Larry Millstein called the 2357th meeting of the Society to order at the Cosmos Club in Washington, D.C. on February 5, 2016 at 8:07 p.m. He announced the order of business and welcomed new members. The minutes of the previous meeting were read and approved. President Millstein then introduced the speaker for the evening, Andrew Ludlow, Project Leader of the Time and Frequency Division at the National Institute of Standards and Technology. His lecture was titled “Improving the Tick-Tock of the Atomic Clock: Telling Time to an Accuracy of One Second in the Lifetime of the Universe”.
Dr. Ludlow began by defining the second: One second is the time it takes for an electron of a cesium 133 atom to transition between its ground state and its excited state just over nine billion cycles. Because time is the physical quantity that we can measure with the greatest precision, we use it as a basis for other units. For instance, the meter is defined as the length of the path traveled by light in a vacuum in approximately one three hundred millionth of a second. The volt is also based on the second, and even temperature and mass have been proposed to be indexed to time.
So, what new windows on the universe does better timekeeping open
for us? One of the most exciting examples is testing for the “gravitational redshift” predicted by General Relativity. This theory states that a clock in a gravitational field should tick slower than one that is not. In other words, an atomic clock in Boulder, CO will behave differently than the same clock at sea level, a mile closer to the Earth’s center of gravity.
General Relativity has not yet been integrated with the other physical forces, and improved atomic clocks are one of our best tools for trying to determine if General Relativity is correct, or if our view of physics as we know it needs revising.
Clocks accurate enough to help investigate General Relativity can also use variations in the Earth’s gravitational field to measure Earth’s topography to centimeter accuracy. This opens up a new ultra-high precision mapping technique known as chronometric geodesy.
Accuracy on the order of one second over the age of the universe also allows us to explore variation in the fundamental constants of nature. Although counter-intuitive, some theories such as string theory appear to require this variation to account for the conservation of energy and other underpinnings of the observed universe. Using atomic clocks based on different elements allows exploration of potential variation in constants such as the fine structure constant and the ratio of proton to electron mass.
Ultra-precise timekeeping may also allow us to finally shine a light on dark matter. Of all the mass in the universe, approximately one quarter is “dark matter” about which we know little. Dark matter interacts only weakly with normal matter, primarily through gravity. It can therefore be expected to have an effect on atomic clocks. If this effect can be detected, it could be used to map dark matter passing through the Earth.
How, then, do we improve our clocks to enable this exploration? Dr. Ludlow explained that all clocks fundamentally consist of an oscillator—that is, a physical generator of periodic activity—plus a counter, which tracks the oscillations. In some atomic clocks, the oscillator is a microwave generator, which is used to illuminate an atom. The electrons of the atom begin in their ground state. If an atom receives a microwave of the correct frequency, an electron will jump to an excited state. The atoms in an atomic clock are thus used as a probe to tune the frequency of the microwave oscillator: if the atoms are excited, the microwaves are being emitted at the desired frequency. This tuned oscillator can then be used to precisely measure time.
The “optical lattice” atomic clock uses a mesh, or lattice, of laser beams to create a standing wave that traps atoms, which can then be closely measured to tune the clock’s oscillator. The high frequency of visible light allows time to be subdivided much more finely than with microwave-based clocks
Beyond the technical improvements, researchers are also developing a catalog of every physical phenomenon that could disturb the natural oscillation of the atoms being measured. Subtle effects such blackbody radiation and quantum tunneling are compiled into an “uncertainty budget” that can then be characterized, mitigated, or accounted for through corrections based on theoretical projections.
Dr. Ludlow explained that international cooperation is critical to improving timekeeping, by permitting the best clocks in the world to be compared and the uncertainty factors better characterized. This painstaking work is producing gradual improvements to one of our most sensitive and versatile tools for measuring the universe.
After the conclusion of the talk, President Millstein invited questions from the audience.
One question was whether ytterbium is the ultimate choice for high precision clocks, or if there could be a better element to use. Dr. Ludlow explained that theory suggests ytterbium is the best element obtainable, but only experiment can tell for sure and this question is actively being investigated.
Another question asked for more information on how so-called “fundamental constants” can vary. Dr. Ludlow acknowledged that this appeared to be an oxymoron, but noted that the universe has changed dramatically over its lifetime, and that conditions in the very early universe may have been subtly different. He noted that even the smallest difference in such constants could make life completely impossible. Thus, whether these attributes are indeed constants takes on something of a philosophical question, in that nothing in physics may require them to be constant…except that if they were different we would not be around to observe the alternate value.
After the question and answer period, President Millstein thanked the speaker, made the usual housekeeping announcements, and invited guests to join the Society. At 9:50 p.m., President Millstein adjourned the 2357th meeting of the Society to the social hour.
Attendance: 67
The weather: Clear
The temperature: 1°C
Respectfully submitted,
Preston Thomas
External Communications Director